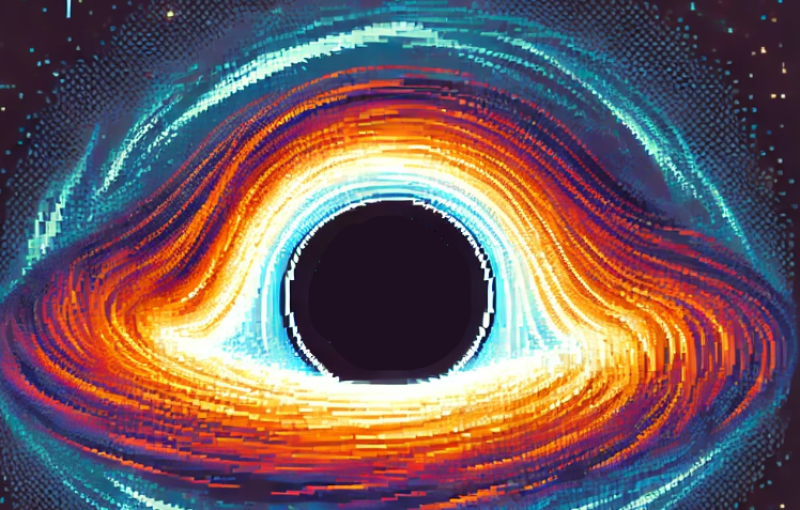
What Happens When Two Black Holes Nearly Collide?
by Jon Scaccia May 22, 2025Two black holes speed past each other in deep space—billions of times heavier than Earth, hurtling faster than bullets. They don’t crash. They don’t merge. They just…swerve.
And in that dramatic cosmic near-miss, they send ripples through the very fabric of spacetime. We call them gravitational waves. And thanks to a surprising mashup of physics fields, we now understand those ripples better than ever.
How? Not by peering through a telescope, but by borrowing techniques from particle colliders like the one at CERN. Yes, scientists just used tools designed for smashing atoms to decode what happens when two black holes nearly kiss.
And the math? It’s not just complicated—it’s laced with the same exotic geometries that live in the hidden dimensions of string theory.
Buckle up. This story is about speed, space, and the strangest math you’ve never heard of.
The Problem: Space is Curved, and So Is the Math
Einstein’s general relativity tells us that mass bends space. When two massive objects—like black holes or neutron stars—pass near each other, their gravitational fields twist their paths like two drunk sumo wrestlers dancing.
This gravitational ballet sends out waves. We’ve only recently had the tools to detect them (shoutout to LIGO and Virgo!). But now, future observatories like LISA will be so sensitive they’ll need predictions with ridiculous precision.
That means scientists must model exactly what happens in these near-collisions, called “scattering encounters.” But here’s the problem: solving Einstein’s equations exactly for two dancing black holes is like trying to untangle spaghetti…blindfolded…underwater…while it’s still cooking.
The Solution: Treat Black Holes Like Subatomic Particles
Here’s where it gets brilliant.
To model these massive cosmic objects, scientists used a framework usually reserved for quantum particles. It’s called Worldline Quantum Field Theory (WQFT), and it lets physicists break the problem into bite-sized pieces using what are basically glorified doodles—Feynman diagrams.
Each diagram is a little snapshot of a gravitational interaction. And at fifth order in Newton’s constant (yes, fifth!), they had to compute 426 of these. Altogether, the calculations took more than 300,000 CPU hours.
Why fifth order? We need this level of detail to match the sensitivity of next-gen gravitational wave detectors. Anything less could cause us to miss the faintest—but scientifically richest—rumbles in the universe.
The Twist: The Math Looks Like Something Out of String Theory
Here’s where things get wild.
To solve these black hole equations, the team had to use incredibly complex mathematical structures called Calabi–Yau manifolds. These aren’t your average geometry shapes. They’re multi-dimensional donuts—sort of. And they’re usually found in string theory, the realm of 10 or 11 dimensions where our universe might be curled up like a cosmic ramen packet.
These shapes show up in the integrals that describe the energy radiated in a black hole flyby. It’s like discovering a unicorn in your backyard while you were just trying to mow the lawn.
This is the first time scientists have shown that the weird, elegant math of Calabi–Yau threefolds is not just for string theorists in windowless offices—it’s needed to understand real astrophysical events.
The Big Takeaway: Precision Modeling Just Got a Superpower
This work delivers a crucial upgrade for gravitational wave predictions:
- The scattering angle (how much the black holes “swerve”) can now be calculated with unprecedented accuracy.
- The radiated energy (how much oomph the gravitational waves carry) includes exotic geometry, but can now be modeled analytically.
- These results match well with expensive numerical simulations—but they’re way faster to compute.
That’s a big deal. Because every time an observatory detects a gravitational wave, it compares the signal to a massive database of “templates.” This research gives scientists sharper, faster, and more accurate templates to spot the weirdest—and possibly most important—cosmic events.
Why This Matters: It’s Not Just Theory
So what’s the big picture?
- If we want to know how black holes form, merge, and scatter—we need better models.
- If we want to understand the early universe or test Einstein’s limits—we need these precision tools.
- If we want to detect weird stuff like cosmic strings, dark matter clumps, or even signals from other dimensions—we need to sharpen our ears to the cosmos.
And it turns out, sharpening those ears means diving into math so strange and beautiful, it could’ve come from a sci-fi novel.
Let’s Explore Together
This isn’t just about black holes. It’s about how creative thinking—from quantum theory to exotic geometry—can unlock secrets hiding in plain sight.
We’d love to know what you think:
👉 What part of this research blows your mind the most?
👉 How would you explain Calabi–Yau manifolds to a friend?
👉 What other cosmic mysteries do you want science to crack next?
Drop your thoughts in the comments, tag a friend who loves space, or share this blog with someone who still thinks math is boring.
Let’s make science irresistible—one black hole at a time.
Stay Updated or Risk Falling Behind
Science is evolving rapidly—and in today’s chaotic information landscape, falling behind means losing ground to misinformation. This Week in Science delivers the most essential discoveries, controversies, and breakthroughs directly to your inbox every week—for free.
Designed for educators and science-savvy citizens, it’s your shield against bad data and outdated thinking.
Act now—subscribe today and stay ahead of the curve.
🔗 Liked this blog? Share it! Your referrals help defend truth and spread scientific insight when it matters most.
Leave a Reply